We have built a machine which can non-destructively identify single, gas-phase molecular ions.
Imagine I give you a single molecule and ask the the most natural question one can ask: what molecule is it? Until October of 2022, we had no method that can answer this question. Today, in our lab, we do. The spectrum below is a vibrational action spectrum of a single tropylium molecule. Our method provides high-quality spectra that are easily sufficient to non-destructively identify single molecules with the added advantage of a high signal-to-noise that enables the measurement of previously unseen transitions.
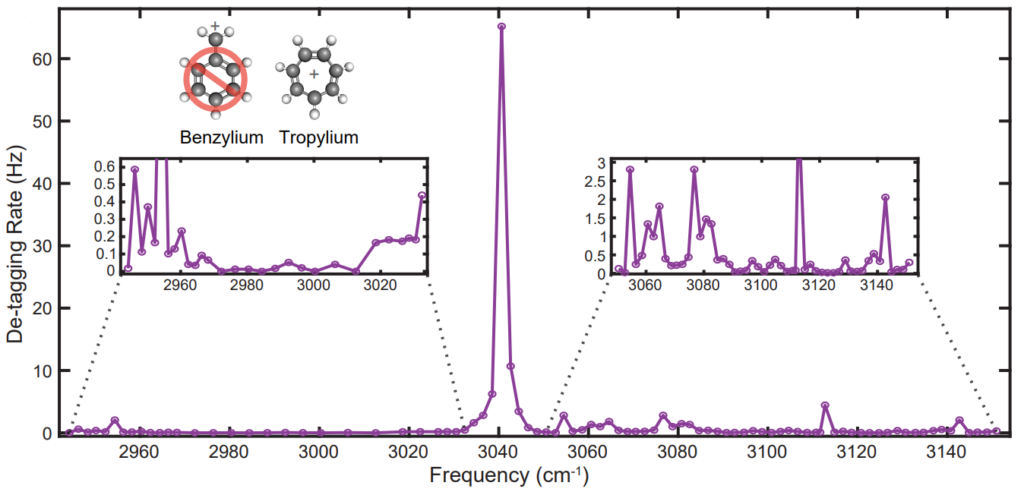
Nondestructive mass spectroscopy using laser-cooled atomic ions is a staple in ion trapping atomic physics groups. Action spectroscopy using inert gases is an established technique popular among analytical and physical chemists. We apply variations of both techniques to create a novel single molecule spectrometer. Extensions of this spectrometer will be able to identify the conformer, stereoisomer of the molecule in question.
The apparatus
The experiment takes place in the linear Paul trap shown below. Ions are deeply confined using a combination of static and oscillating electric fields. The deep confinement allows us to study a single molecular ion for days at a time. Everything is kept at cryogenic temperatures (around 8K) and under ultrahigh vacuum, meaning the molecular ions are well localized in a pristine & well-controlled environment.
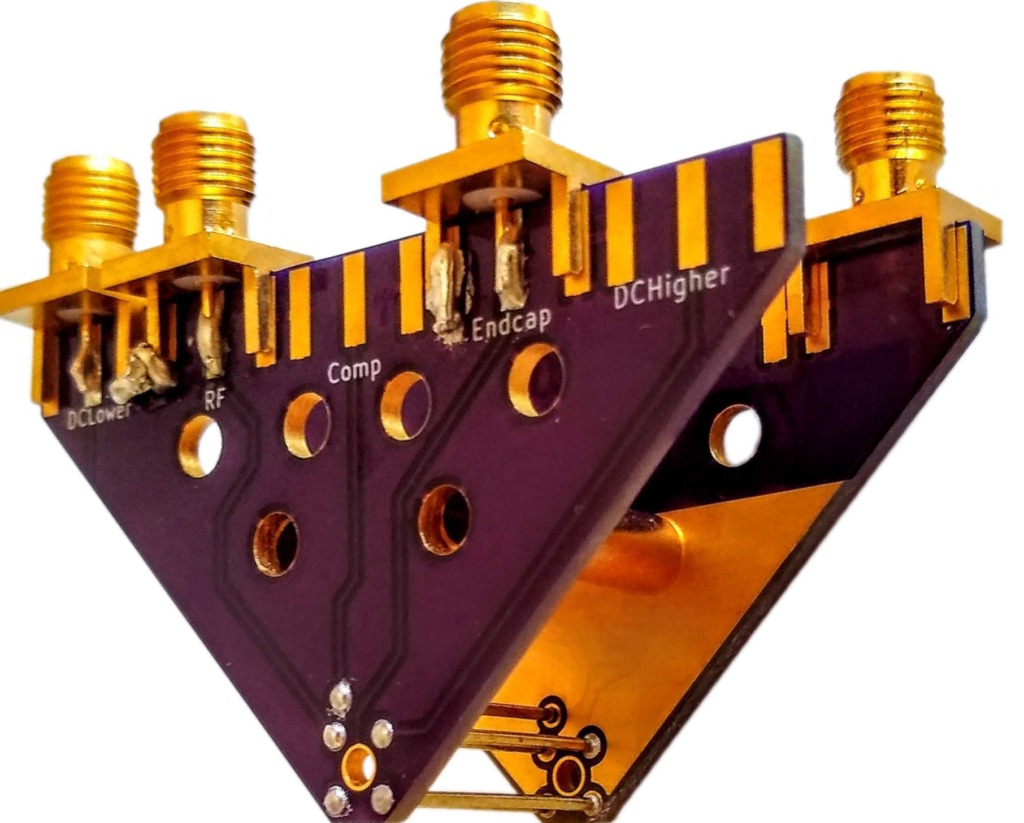
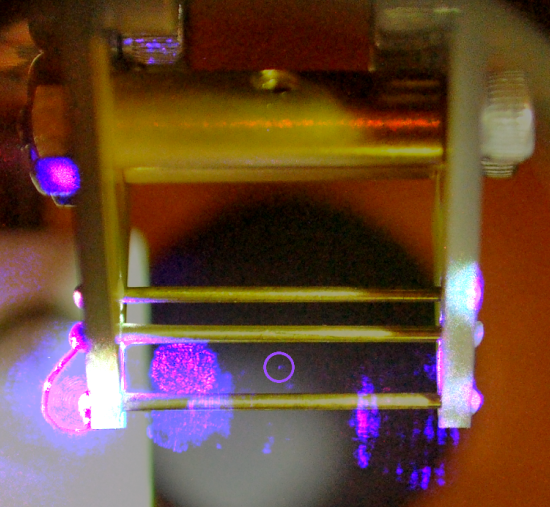
How does our spectrometer work?
We cannot directly see the molecule in question, so we exploit tricks developed by atomic physicists. Atom are simple and have a well-understood electronic structure. We can trap an atomic strontium ion and use a violet (422 nm) laser to cool the atom to mK temperatures. As a bonus, the atom fluoresces and we can directly image it.
Molecules are more complicated. They have electronic structure like the atom, but also vibrate and rotate. There is generally not a set of lasers that can laser cool a molecule and make them fluoresce. Instead, we must trap both in the same trap and use the atomic ion as a reporter to tell us about changes in the molecule. Nondestructive mass spectrometry is the method that allows us to detect changes to the molecule.
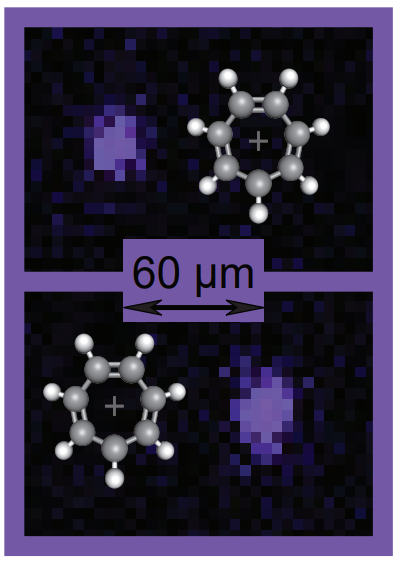
Nondestructive mass spectrometry
Most methods that work with molecular ions are destructive. The molecules produce a signal at the detector, but are lost in the measurement process. Here, we rely on the ion trap to measure the mass without losing the molecule.
The strontium atom and molecule are co-trapped. Though not chemically bonded, the two share a strong Coulomb interaction due to their charge. Like a coupled harmonic oscillator, we can describe the motion using two modes (shown below for two Sr+ trapped together). The frequency of the modes depend on the masses of the trapped species. We can easily measure the frequency simply by observing the fluorescent Sr+, which allows us to measure the mass of the molecule completely nondestructively.
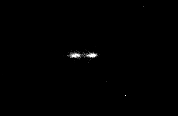
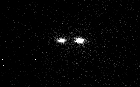
Translating a mass measurement to a spectrum
We can apply an inert gas “tag” to the molecule to indicate the absorption of a photon on resonance with a molecular transition. Nitrogen (N2) is a robust tagging species that we typically use, though hydrogen and neon are also possible. The tag adheres weakly to the molecule by the Van der Waals force. When an infrared laser is tuned to resonance with a molecular transition to an excited vibrational state, the molecule vibrates and “shakes off” the tag. Since the “shake-off” changes the mass of the molecule by 28 amu, we can easily detect when such an event occurred.
Why is single molecule spectroscopy important?
An obvious application for the spectrometer would be in the analysis of rare samples. For example, the Cassini Probe detected a few molecules originating from Enceladus. The measurement was destructive and the identity of the molecules is not exactly known. Though our experiment is a long way from such an application, a variation of our technique could prove valuable in the analysis of rare samples collected in space.
The ultra-long lifetime of the molecular ion in the trap gives us exceptional signal-to-noise and has allowed the measurement of previously unobserved weak transitions. We believe these are combination bands and should provide an experimental test to state-of-the-art quantum chemical computations.
Another possibility is testing chemical reactions at the single molecule level. The idea behind this is to measure the spectrum of a single molecule & confirm it’s identity, then add a reactant. After the reaction, the product will remain trapped as long as the mass is within about 50-250 amu. We can then take the spectrum of the product and confirm it’s identity. This platform would enable the study of reactions relevant to astrochemistry, combustion, and solvation chemistry.
A tagged tropylium molecule
How do we go beyond tagging spectroscopy?
Tagging spectroscopy has long been the workhorse for obtaining a vibrational spectrum of molecular ions. It is robust technique, however it does suffer some downsides.
For one, the spectrum obtained is the of the Van-der-Waals complex (tropylium-N2 complex is described above), not of the bare molecular ion. The weakly bound tag does perturb the vibrational levels slightly and adds additional complication to interpreting spectra.
Another downside is the weakly bound tag quickly dissociates under infrared excitation. The short predissociation lifetime limits the resolution afforded by tagging spectroscopy and generally precludes rotational resolution.
Fortunately, the Schlemmer group in Köln recently demonstrated a robust alternative to tagging spectroscopy. Vibrationally excited molecular ions can undergo inelastic collisions with cold buffer gas atoms where vibrational energy is converted to translational energy. These energetic molecules are ejected from the trap where they are detected.
We recently demonstrated a straightforward non-destructive adaptation to record the vibrational spectra of a single molecule. In this approach, an inelastic collision with a vibrationally-excited molecular ion imparts enough kinetic energy on the molecule to “heat” it out of the Coulomb crystal.
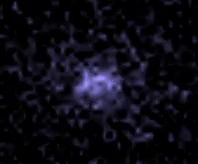
We simply measure inelastic events as a function of mid-IR frequency to construct a spectrum of the molecular ion.
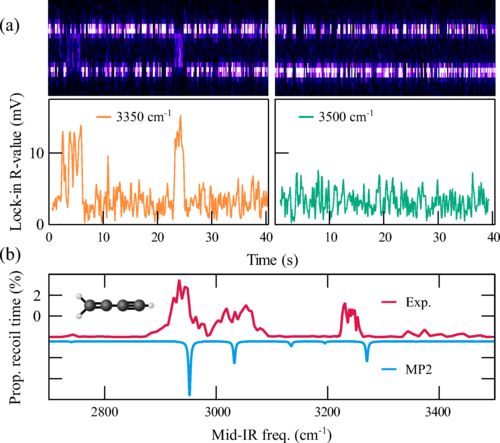
Is inelastic recoil spectroscopy better for single molecule analytics?
Inelastic recoil spectroscopy allows data acquisition on a faster timescale compared to tagging spectroscopy. Tagging spectroscopy requires a high density of buffer gas to adhere the van-der-Waals tag. The large amount of background gas takes time to pump out to allow the Coulomb crystal to re-cool and also inadvertently causes unwanted reactions with trace contaminants. Without the need for these gases, inelastic recoil spectroscopy has allowed us to trap & study a single molecule for a month at a time!
Crucially, we should be able to obtain rotational resolution with this new method. This is key for future precision-measurements & the determination of a molecule’s chirality. Rotational resolution gives much more insight into the structure of a molecular ion.